Ice Sheet Dynamics
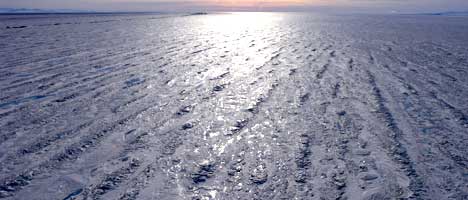
Contacts, for more information:
The present-day ice sheets contain the equivalent of about 65 meters of global sea level rise, locked in the form of ice. Consequently, even relatively minor changes in the imbalance of the ice sheets have global significance. Two decades of satellite measurements in Greenland and Antarctica reveal snapshots of ongoing ice-sheet changes, which indicate potential significant sea-level rise over the next centuries. In order to better understand and constrain the projections of how climate will alter ice sheet evolution and how changes in the ice sheets will influence global climate change in the coming centuries, we need to understand the dynamics of ice sheets and the way ice is discharged from the ice-sheet interior to their margins — into the surrounding oceans.
Ice sheets flow under their own weight. The rate of ice-sheet flow is determined by the balance between the effects of gravity, basal resistance opposing the ice sheets, and internal deformation of ice. Depending on basal resistance, which is determined by the nature of underlying strata (e.g., hard rock, deformable sediments, etc), ice flow can be dominated by either basal sliding (as happens in ice streams) or vertical shear (as happens in parts of ice sheets where ice is frozen to the bed).
GFDL Research
Stability of marine ice sheets
Ice sheets that rest on bedrock below sea level are called marine ice sheets. The transition between the grounded part of marine ice sheets and their floating parts (ice shelves) is the grounding line. Dynamics of the grounding line determines the horizontal extent of marine ice sheets. The ice discharge across the grounding line from the grounded part of the ice sheet to the floating part, ice shelves, determines ice-sheet contributions to the global sea level. An existing marine ice-sheet instability hypothesis suggests that the stability of marine ice sheets depends only on the local bedrock slopes. GFDL scientists have developed a theory of laterally confined marine ice sheets (Haseloff and Sergienko, 2018). This theory suggest stability of the grounding line of such ice sheets is determined by the local properties of the bedrock and the integral properties of the ice shelves. These results indicate that the marine ice-sheet instability hypothesis is not valid for marine ice sheets flowing into laterally confined ice shelves.
Ice-Stream Dynamics
Ice streams are regions on ice sheets that move significantly faster than the surrounding ice. They act as ways for ice to discharge from the interior of the ice sheets to the margins, at rates controlled by conditions at the ice-bed interface. The basal resistance of ice streams is highly variable, but the causes of this heterogeneity are not well understood. Mostly, it is attributed to changes in the underlying geology, such as variations in the sediment thickness.
Figure 1. Inferred basal conditions under Thwates (left) and Pine Island (right) glaciers overlayed on satellite images of these glaciers taken by MODIS
Direct observations at the glaciers bed over extensive areas to understand the basal mechanics of ice flow is logistically infeasible. An alternative approach is to employ inverse methods and utilize surface observations to infer the spatial distributions of basal resistance of ice streams. GFDL scientists have applied inverse models together with high-resolution data sets of satellite and airborne collected observations to two Antarctic ice streams, Pine Island and Thwaites. Both are currently losing mass at an accelerated rate. This work has revealed that the basal traction underneath these glaciers has organized spatial patterns such that narrow, rib-like structures with very high basal shear stress are embedded in much larger areas with zero basal shear. Such an organized spatial pattern implies that it arises from the complex interactions of glacier flow, subglacial water and deformable sediments.
The basal traction ribs most likely arise from the long-term evolution of dynamic instabilities, manifesting as bed-friction variation arising from changes in the effective pressure in space (the difference between ice overburden and subglacial water pressure). The present spatial configuration of basal resistance in these ice streams is not immutable and can potentially change over decades to centuries in response to changes to ice-sheet geometry or water input. Changes in basal shear stress distributions in the vicinity of the grounding line inevitably cause variations in ice flow and its flux through the grounding line. This triggers its migration, with consequent changes in ice discharge to the ocean, and the glaciers’ contribution to sea level.
Ice-Shelf Dynamics
Figure 2 Larsen B Ice Shelf before (top) and after (bottom) collapse. (MODIS images from NASA’s Terra satellite —National Snow and Ice Data Center, University of Colorado, Boulder)
Ice shelves are floating extensions of ice sheets. A spectacular collapse of Larsen A and B ice shelves (Antarctic Peninsula) in 1995 and 2002, respectively, introduced a completely new timescale to glaciology. The Larsen B Ice Shelf lost an area of 3,600 square kilometers (approximately the size of Rhode Island) that disintegrated into numerous icebergs within three weeks. For comparison, large tabular icebergs originating from the Filchner-Ronne and Ross ice shelves usually calve only about twice a century.
An increasing number of surface lakes and melt ponds on the surface of the Larsen A and B ice shelves were detected in satellite images during several austral summers prior to their disintegrations. The presence of lakes indicated that these ice shelves were experiencing strong surface melting. Several thousands of these lakes synchronously drained a few days prior to the Larsen B collapse. Satellite observations revealed a number of small scale icebergs. A unique triggering mechanism is required to explain both a synchronous drainage of surface meltwater and specific spatial scale of icebergs. Considerations of ice-shelf deformation in response to the presence (filling) and the absence (drainage) of surface lakes suggest that stresses induced at the ice-shelf surface in the immediate vicinity of a filled lake and in the center of a drained lake are sufficiently large to initiate fractures and crevasses. Upward propagating fractures from the ice shelf base meet downward propagating fractures from the ice shelf surface, leading to through-cutting rifts.
The spacing of the fractures is determined by the spatial distribution and depths of lakes. Analysis of the lake geometries show that the spacing of these fractures caused a large proportion of the Larsen B Ice Shelf fragments to have aspect ratios that were unstable to capsizing. Moreover, the event that leads to lake-induced fractures can be self-catalyzed and self-sustaining and can cooperatively occur across widely varying flow and stress regimes of the Larsen B Ice Shelf.