May 4th, 2012
Key Findings
- Regions that are generally characterized by subsiding air on the large-scale (e.g. the Eastern Subtropical Pacific) experience a reduction of low cloud in the presence of absorbing aerosol that is primarily attributable to “cloud burning” dictated by a negative correlation between atmospheric temperature and relative humidity.
- Regions of deep convection (e.g. the Western Pacific Warm Pool) experience an increase in mid-level cloud amount, which may be due to the horizontal detrainment of cloudy air forced by absorbing aerosol heating in the mid-troposphere, but also appears to be dependent on the model’s cumulus parameterization.
- The tropospheric response over subsiding regions appears to be largely the result of first-order physics that is expected to be transferable across different models, while the response over convective regions appears to result from a mixture of physical and nonphysical mechanisms. Care should, therefore, be taken when including the tropospheric response to absorbing aerosols in calculations of its radiative forcing over convective regions.
- For the majority of signals, however, radiative flux perturbation can be expected to be a physically robust measure of absorbing aerosol radiative forcing for AM2.1.
Geeta Persad, Yi Ming, and V. Ramaswamy. Journal: Journal of Climate. DOI: 10.1175/JCLI-D-11-00122.1
Summary
An ongoing challenge in quantifying aerosols’ impact on the climate is determining an optimal way of calculating aerosols’ radiative forcing. For absorbing aerosols, in particular, studies have shown that a forcing calculation that does not include the tropospheric response to absorbing aerosol (instantaneous forcing) is a poor proxy for the change in global mean surface temperature caused by the aerosol.
Radiative flux perturbation, a forcing calculation that does include the tropospheric response, is a much better temperature proxy, but runs the risk of incorporating nonphysical processes. In this paper, we investigate the physical mechanisms behind the tropospheric response to absorbing aerosols in GFDL’s AM2.1 Atmospheric General Circulation Model in order to shed light on whether it may be robustly included in the calculation of absorbing aerosols’ radiative forcing.
We conduct a series of highly idealized experiments in which AM2.1 is run with a globally uniform layer of black carbon, and compare the top-of-atmosphere fluxes with those of a pre-industrial control run after the troposphere has been allowed to respond to the aerosol. By analyzing where differences exist between these fluxes and those that occur before the troposphere responds, we can better understand what tropospheric processes would be incorporated into a radiative flux perturbation calculation and whether they are physically robust.
The tropospheric response manifests primarily in cloud changes and differs based on the large-scale environment. Over regions where the large-scale air is subsiding (sinking), absorbing aerosol heating near the boundary layer top causes a substantial reduction in low cloud amount, both thermodynamically (by decreasing relative humidity) and dynamically (by lowering cloud top).
Over convective regions, on the other hand, heating above the boundary layer hinders the vertical development of deep cumulus clouds, causing horizontal detrainment of cloudy air and stronger cloud reflection. However, much of the convective cloud change appears to be dependent on the model’s cumulus parameterization. This mechanistic understanding of the tropospheric response allows us to better characterize the physical robustness of forcing values derived from the radiative flux perturbation over different regions.
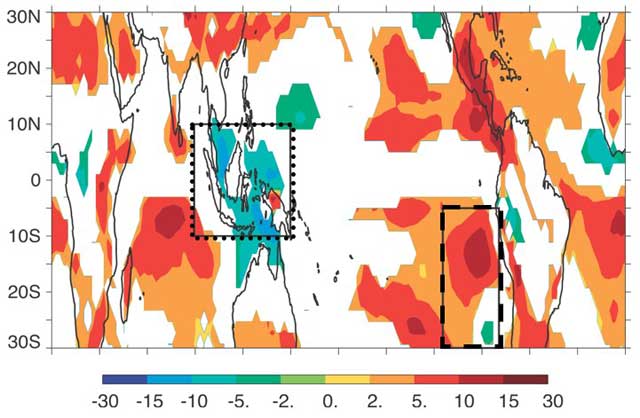