Radiative Forcings
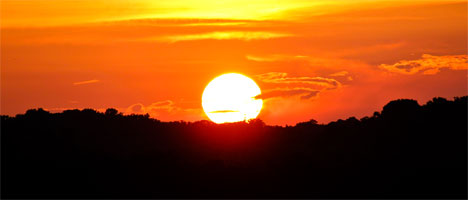
Contacts, for more information:
Atmospheric radiative transfer is the science of understanding how electromagnetic radiation emitted by both the Sun and Earth interacts with the gases, clouds and particles making up our atmosphere. The changes in energy due to these interactions are responsible for many variations in temperature and weather that we experience in everyday life. For example, cloudy nights are normally warmer than clear nights because of radiative transfer processes, with the nighttime clouds reducing the energy lost to space by the surface of the planet. The science of radiative transfer also explains a wide range of beautiful natural events, such as rainbows, moon halos, sundogs and the color of the sunset. On longer timescales, the processes by which energy leaving the Earth is altered by increased atmospheric greenhouse gases, such a H2O and CO2, is explained by radiative transfer.
In a general circulation model (GCM), radiative transfer translates changes in atmospheric constituents, such as clouds, aerosols, water vapor, and CO2, into changes in energy reflected, absorbed or emitted by the atmosphere. Accurate radiative transfer calculations are therefore required to model how the global climate system will be altered in response to changes in both anthropogenic and natural emissions.
GFDL Research
In order to have the most accurate and up-to-date radiative transfer science in our GCMs, we are carrying out research that varies from incorporating improved understanding of water vapor spectroscopy (Paynter and Ramaswamy, 2012) to using global observing systems to validate our GCM energy balance at the surface (Freidenreich and Ramawamy, 2011). Understanding the radiative transfer of the stratosphere and how this impacts global temperature is also another area of research expertise at GFDL (Schwarzkopf and Ramswamy, 2008, Austin et al., 2013).
Our main objectives are to maintain radiative transfer codes for usage in GFDL models; to maintain a line by line radiative code; to make use of the latest theoretical and measurement data within the radiation codes; to benchmark and test our radiation codes against other codes and measurement data; and to actively participate in radiative transfer inter-comparison projects. Our ultimate goal is to understand and evaluate the impact of radiative transfer upon climate simulations.
The flux per a wavenumber calculated for the CIRC case 2 atmosphere, as modeled by the RFM line by line code.
Research Highlights